A Bridge to the Quantum World
On the optical tables in physicist Monika Aidelsburger’s laboratory, an elaborate landscape of lenses, lasers, mirrors and optical fibers is in the early stages of its “evolution.” At the moment there are still relatively few elements, but her experiments are highly complex and she will soon need to accurately position and calibrate many delicate components. This makes the assembly of the final set-up extremely time consuming.
Immense care and painstaking precision are essential when it comes to modeling quantum phenomena with neutral atoms in optical lattices. Optical lattices are formed by intersecting laser beams that interact to produce a network of “potential wells.” Ultracold atoms can be trapped in these wells like eggs in a preformed carton. In this case the particles of interest are ytterbium (Yb) atoms, which have been cooled to temperatures very close to absolute zero.
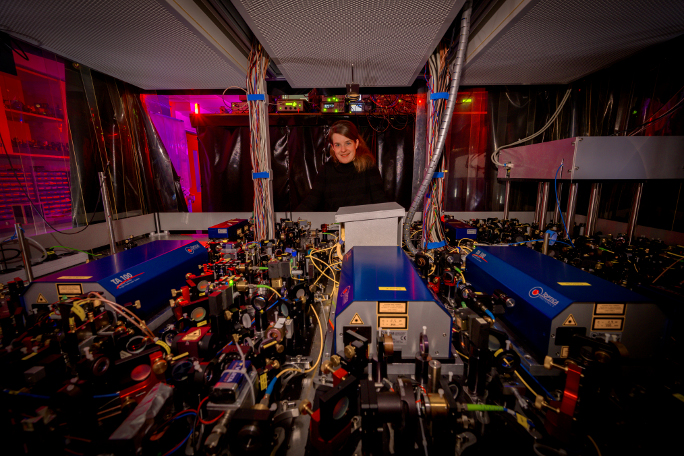
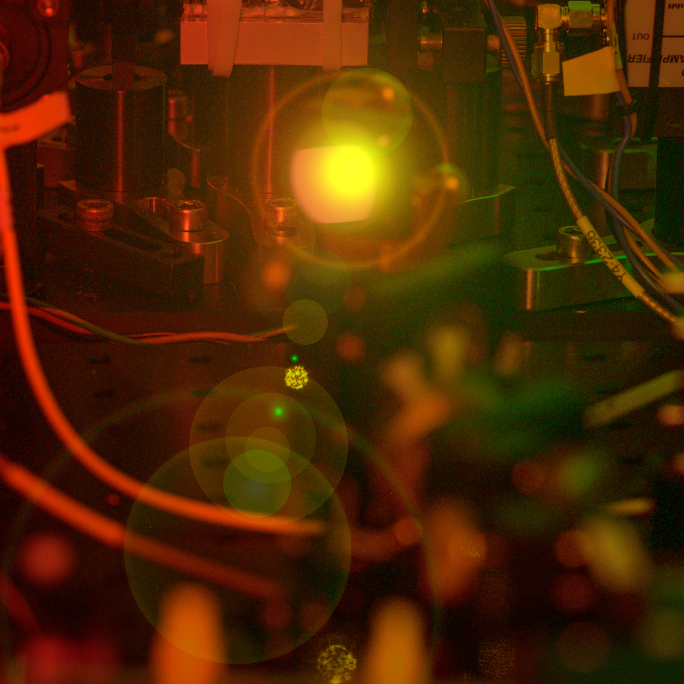
Local Control of Single Particles
Last year, Aidelsburger received a generously endowed grant from the European Research Council (ERC), giving her the opportunity to develop a novel type of optical lattice in which each cell can be individually addressed. This would allow her to locally control the motion of the atoms trapped in the lattice, opening the door to a whole class of novel model systems that go beyond traditionally studied solid-state systems. “With this new experiment, we hope to develop methods that enable us to study fundamental interactions between elementary particles,” she says.
In recent years, Aidelsburger has built up a research group of her own at LMU to explore phenomena in quantum physics, whose exact nature remains obscure. Most of these enigmatic phenomena involve large numbers of strongly interacting particles, which effectively makes their behavior inaccessible to a detailed theoretical analysis. This is where quantum simulations come into play—they offer an experimental way to find the answers that continue to elude theorists.
It is fascinating to consider how much progress has been made over the last few years in the control of single particles.Prof. Dr. Monika Aidelsburger, Group leader, Quantum Optics Group at LMU Munich
Benefits of Collaboration in the New Cluster
To find the optimal configuration for her next experiment, Aidelsburger will take advantage of tricks and techniques developed by her colleagues in Professor Immanuel Bloch’s department at LMU to help them uncover the secrets of the quantum world. “We collaborate closely with the other laboratories, and we benefit greatly from the discussions and the exchange of expertise and insights in the Faculty of Physics and in the new MCQST Cluster,” she explains. The Munich Center for Quantum Science and Technology receives funding within the Germany-wide Excellence Strategy.
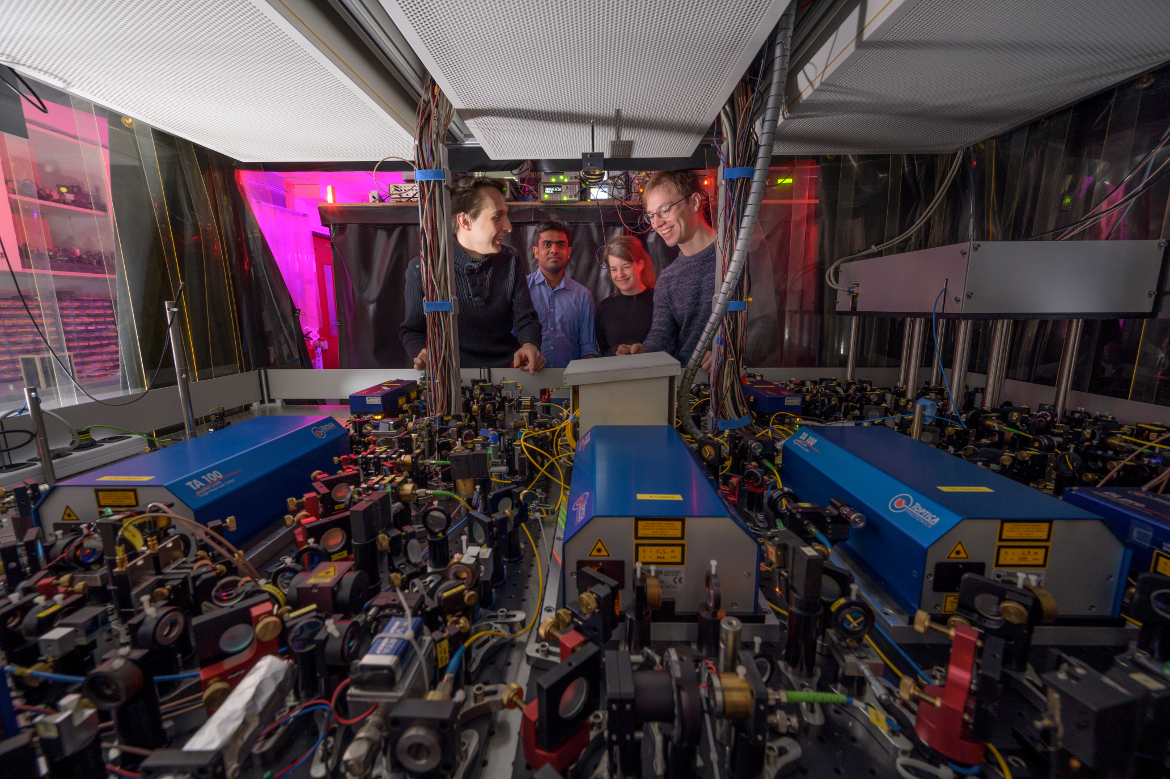
Trapped in Light
Ultracold atoms trapped in optical lattices serve as useful models for investigating the physics of condensed matter under controlled conditions. Interactions between the atoms can be controlled by altering the depth of the potential wells, which is normally set globally for the lattice as a whole. Aidelsburger wants to use locally controllable lattices to simulate “lattice gauge theories,” which describe the interactions between matter and “gauge fields.” These theories make use of (fictitious) lattices in which the “vertices” represent the “matter” particles, while the “links” that connect them model the strength of the interactions between them, which are mediated by “gauge” particles.
A New Way to Elucidate Quantum Phenomena
The main attraction of this approach in the simulation of lattice gauge theories is that it promises to provide access to new areas of research and uncover links between different disciplines of physics. Because gauge theories play a fundamental role in so many fields of physics, they provide a natural bridge between these different domains. Such theories are used to describe both the physics of strongly interacting electrons in solid-state systems and important aspects of quantum electrodynamics. In this sense, they have the potential to provide a unified picture of a wide range of quantum phenomena—which might lead to new ways of probing and elucidating quantum phenomena that have so far remained inaccessible to experimental tests.
“Young Scientists Need Longer-Term Perspectives”
The prospect of working on such an exciting and challenging project as a tenure-track professor in Bloch’s department no doubt played a role in Aidelsburger’s decision to return to Munich. Prior to this, she had served a stint as a postdoc at the Collège de France in Paris. “Young scientists need longer-term perspectives, especially when the experiments involved are as complicated as they are in this case,” she points out.
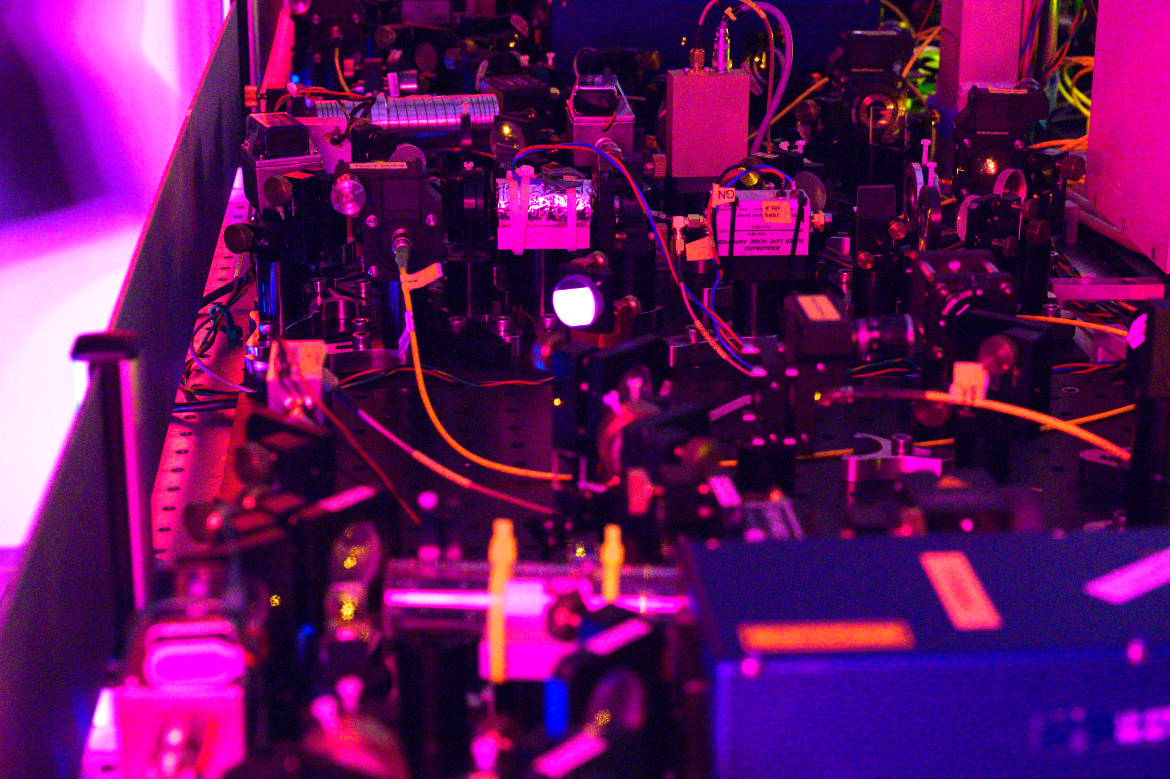
Step by Step to Higher Dimensions
Aidelsburger’s particular interest lies in simulating the effects of magnetic fields on quantum systems. “Magnetic fields can be described with the help of gauge theories,” she explains. But implementing such simulations requires an extremely complicated experimental set-up. She chose to work with ytterbium atoms because they can exhibit two different energy states, both of which are long-lived. This makes it possible to encode the “matter” and “gauge” particle degrees of freedom using the two internal states. It is possible (although very difficult to achieve in practice) to manipulate the states in such a way that the motion of atoms in different energy states is coupled.
We collaborate closely with the other laboratories, and we benefit greatly from the discussions and the exchange of expertise and insights.Prof. Dr. Monika Aidelsburger, Group leader, Quantum Optics Group at LMU Munich
“It’s this kind of local coupling that enables such a system to be used to experimentally implement the basic building blocks of lattice gauge theories,” says Aidelsburger. Once this is accomplished, it should be comparatively easy to extend such a model to larger lattices and higher dimensions. “It is fascinating to consider how much progress has been made over the last few years in the control of single particles,” she says.
The capacity for local control is indispensable for this kind of experiment. And the research environment and infrastructure in Munich, including the MCQST Cluster and collaborations with the Max Planck Institute for Quantum Optics in Garching, provides an ideal basis for this work. “There is nothing comparable anywhere else in Germany,” says Aidelsburger.