Quantum Mechanics 2.0
Joachim von Zanthier and Stefan Richter are focusing intently on a monitor. They have placed tiny nanoparticles, known as quantum dots, under a microscope, and are illuminating them with pulsated laser beams. A camera with a highly sensitive CCD sensor sends the images directly to a computer. What makes this experiment special is the fact that the light scattered by the quantum dots is not used directly to form the image. Rather, the image only becomes apparent after the multiplication of individual pixel values from the camera, known as intensity correlations. “We assess each one of these snapshots individually, and we can pinpoint the location of each quantum dot, as well as certain atoms, in our samples from the correlation of the photons,” explains von Zanthier.
Quantum Imaging
Photon Pulses Unlock Molecular Structure
This method of incoherent diffractive imaging (IDI) was developed at FAU by the Quantum Optics & Quantum Information working group (QOQI) led by von Zanthier. Unlike traditional methods of X-ray crystallography where incoherent light is detrimental, incoherent light is central to the experiments conducted by researchers specializing in quantum optics. When photons are directed at atoms, these atoms scatter off fluorescent light, resulting in diffuse noise. “It is this noise, the incoherent light, that is central to our analyses,” explains von Zanthier.
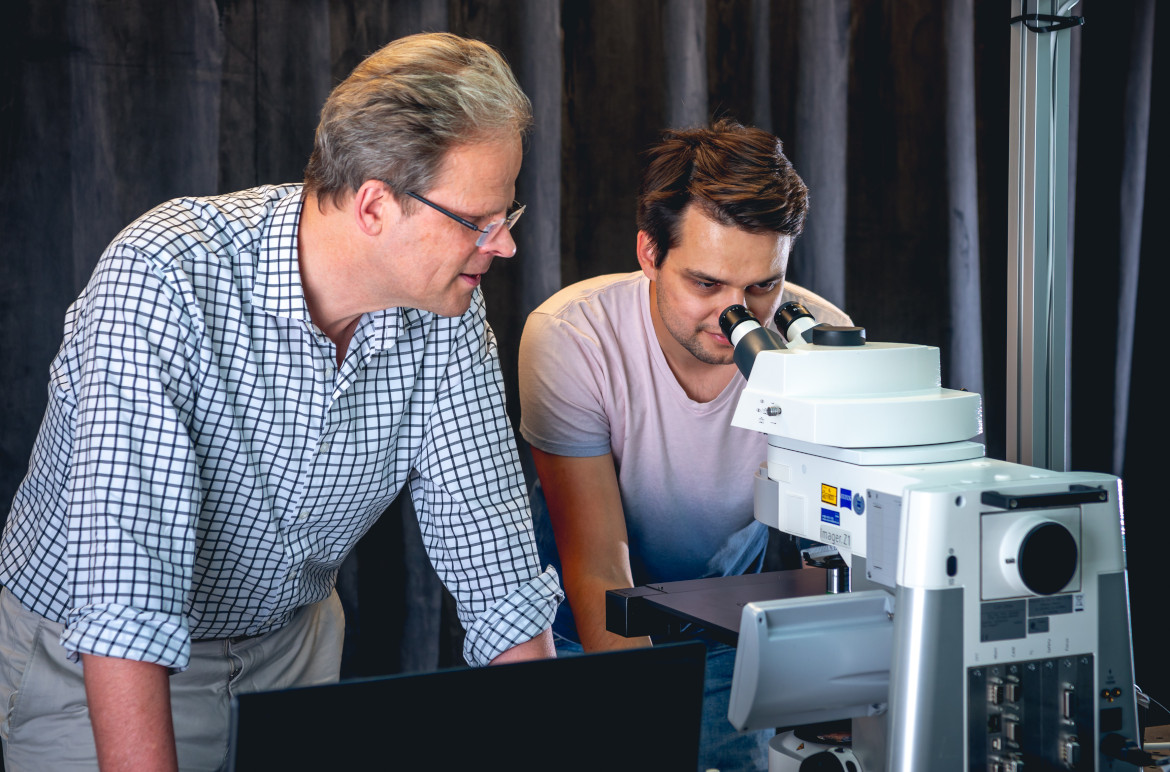
The researchers hope that IDI will enable them to obtain X-ray images with a considerably improved resolution. To do so, they need X-ray pulses in the femtosecond time scale, but these can only be produced by free electron lasers. There are only a handful of these lasers in the world. One of them is at Deutsches Elektronen-Synchrotron DESY in Hamburg, and the researchers from Erlangen work closely with their colleagues from the north.
Promising Applications in Biological and Medical Research
The IDI procedure is particularly promising for biological and medical research. One advantage is that fluorescent light can be used in the X-ray range to better determine the position of atoms. As each type of atom radiates a specific fluorescent light, each type of atom can be made visible individually in complex molecules or proteins. This is not possible with the coherently scattered X-ray light that has been used until now.
Today, we are carrying out simulations using nanocrystals, but in just a few years’ time we hope to apply our findings to individual cellular components or muscle fibers.Prof. Dr. Joachim von Zanthier, Experimental Physicist at FAU
Another advantage is that incoherent light in the visible range can penetrate deep layers of skin but still be used for imaging purposes, which cannot easily be done using standard microscopy. The group currently conducts simulations using nanocrystals, with the hope that they will be able to apply their findings to individual cellular components or muscle fibers in the next few years. “Looking to the future, examinations will even be able to be carried out in living organisms,” von Zanthier adds.
World-Leading Research in Quantum Metrology
Quantum imaging as described above is only one aspect of quantum metrology, an area in which FAU is doing world-leading research. Other working groups at FAU and the Max Planck Institute for the Science of Light also focus on using phenomena of quantum mechanics to conduct highly sensitive measurements of physical quantities. In ghost imaging, for example, entangled pairs of photons are transmitted and detected in separate beams, allowing high resolution images to be recorded under difficult conditions, such as in cases where there is a lot of noise. Quantum sensing involves precisely manipulating the interaction between light and atoms in microcrystals in order to accurately detect spatially resolved magnetic fields. The potential benefits are considerable, especially in fields such as medicine or geodesy.
Quantum Computing
Toward Real-World Applications
Quantum mechanics is shifting away from purely fundamental research and into real-world applications, in what is often referred to as the second quantum revolution. The most well-known example is the quantum computer, which challenges our understanding of classical binary computing operations. “Quantum computers store information in quantum states,” explains Michael Hartmann. “One qubit, the basic processing unit, not only assumes the value of zero or one but can maintain both states simultaneously. The values assumed by the qubits are only measured after the computing operation.”
We cannot begin to imagine the number of possible combinations quantum computers can calculate. We’re looking for codes that make the process more effective.Prof. Dr. Michael J. Hartmann, Physicist at FAU
Hartmann was a member of the international Google team that developed Sycamore, the first quantum computer that has been proven to have completed a complex computing task. The processor, which consists of a matrix of only 54 qubits, needed just three and a half minutes to complete the task. The fastest supercomputer in the world would have taken approximately 10,000 years to solve the same problem. “What makes quantum computers so powerful is the exponential increase in computing power that doubles with every qubit,” Hartmann explains. “A quantum chip with 300 entangled qubits can assume more states than the number of atoms in the universe.”
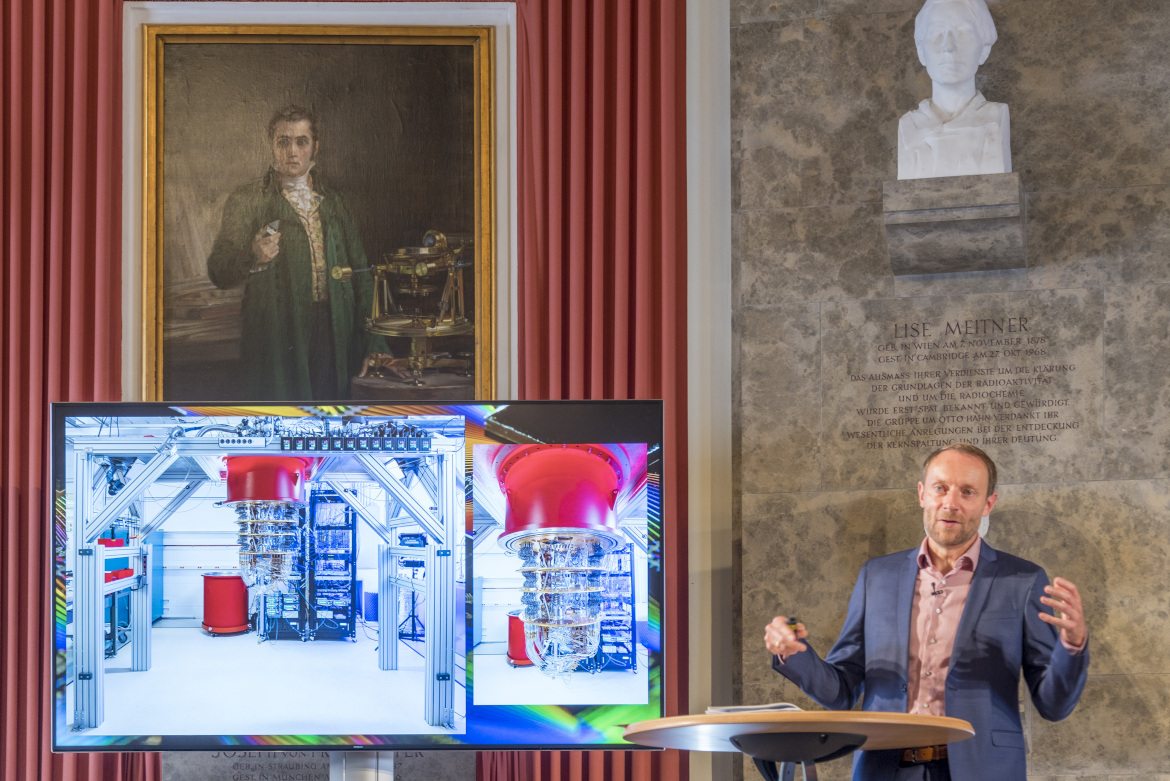
Advances in Quantum Computers
Hartmann, a professor in theoretical physics at FAU, has now established his own research group at FAU, dedicated to furthering the development of quantum computers. One of their major goals is to make qubits more robust and less liable to error by shielding them better from each other and from external influences. “The Earth’s magnetic field has a disruptive effect—even cosmic rays are a problem,” states Hartmann. One of the concepts being pursued by the group involves packing qubits in individual aluminum chambers which still allow them to connect to each other effectively. Another approach involves the possibility of using targeted interferences to prevent disruption from magnetic fields.
The team is also investigating software, such as new algorithms that can cut the required number of quantum gate operations. As Hartmann puts it, “Quantum computers are advancing into areas offering a mind-blowing number of possible combinations, such as simulations of the quantum behavior of electrons and atoms, or modular construction systems in industry. It would be interesting to discover whether the quantum chip has to calculate all possibilities or whether codes could make the process more effective. These are the codes we are looking for.”
Quantum Cryptography
Ensuring Secure Encryption
The massive potential of quantum computers may also have a significant impact on secure communications. Their sheer power is breathtaking, but the risks are significant. “We need to start thinking about how we can protect data from access by unauthorized parties. Just because they are secure today does not mean they always will be,” says Christoph Marquardt. “Conventional encryption is based on complex mathematical calculations. However, if quantum computers are used in future, the methods generally used today will quickly become outdated and open to security breaches.”
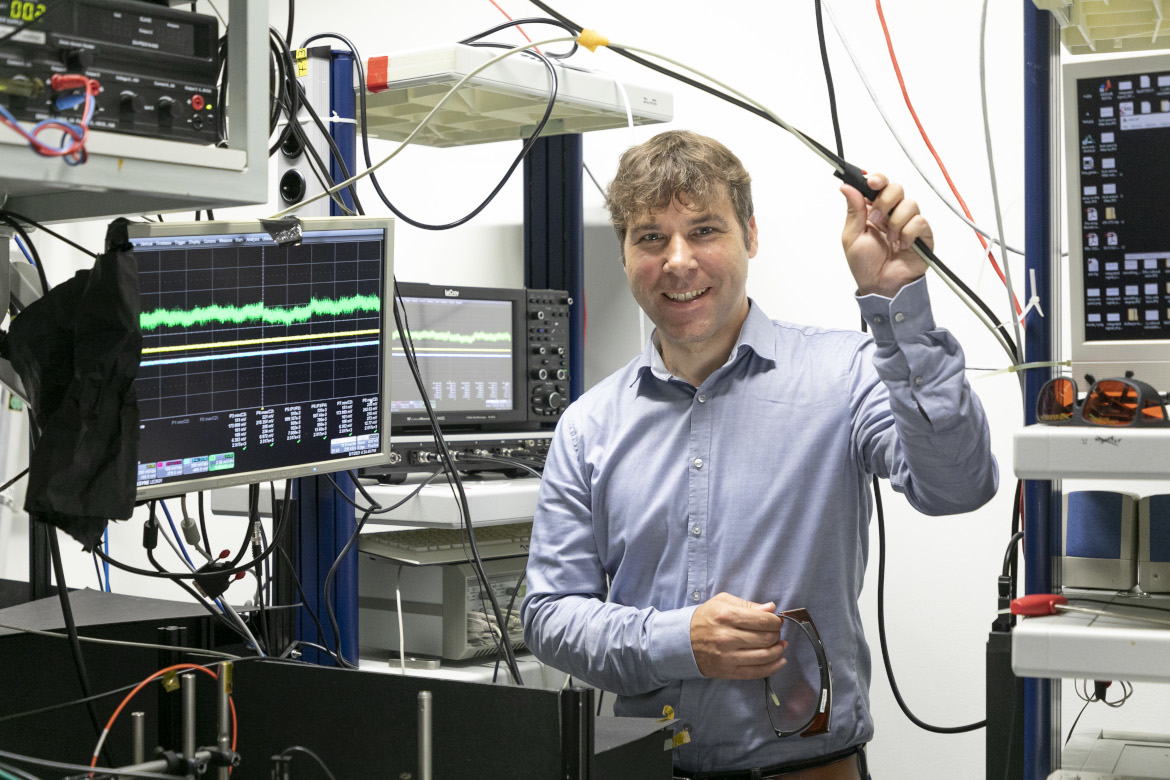
Marquardt leads a research group at the Max Planck Institute (MPI) of the Science of Light in Erlangen, which is designing new methods of encryption based on quantum mechanics. The principle is just as fascinating as it is difficult to grasp: sending and measuring quantum states of light makes it possible to discover whether encrypted information has been manipulated or tapped into. “By sending many different quantum states to the recipient, we can subsequently measure whether a third party has managed to tap into the data,” explains Marquardt. “Each time data are accessed, the quantum states change. Monitoring this enables us to detect any unauthorized access. The measured data can be used to create a private key, which can subsequently be used for encryption purposes.”
We are shaping the transition to quantum mechanics 2.0. Physics, computer science, mathematics: each one has a valuable contribution to make.PD Dr. Christoph Marquardt, Experimental Physicist at FAU at the Max Planck Institute for the Science of Light
With its research into quantum encryption, the Erlangen team is part of the Federal QuNET initiative which funds the development of secure and robust IT networks against the cyberattacks of tomorrow. IT security is a particular challenge in our increasingly connected world, and affects all areas of our modern lives: administration, governments, the banking sector, the health sector, and last but not least, the Industrial Internet of Things (IIoT). The group is also involved in the QUBE project, funded by the Federal Ministry of Education and Research, which focuses on distributing quantum keys by satellite. Within the context of this project, scientists at the MPI in Erlangen are building a quantum random number generator, which will be launched into space in 2022 in order to be tested under real conditions.
Shaping the Transition to Quantum Mechanics 2.0
However, the Marquardt group is not only concerned with data security—it also focuses on communication infrastructure. “Even if it is still a few years down the road, at some point the question will be raised of how to connect quantum computers,” Marquardt explains. “Quantum states are fragile and cannot be transferred directly over long distances.” Researchers are investigating which methods could prove suitable for transmitting information. One solution could be intermediate mini quantum computers.
“We are living in exciting times, in which we can shape the transition to quantum mechanics 2.0,” says Christoph Marquardt. “Whilst researchers one hundred years ago focused predominantly on the foundations of particle physics, today we are dealing with applications based on chance and probabilities. The special thing about our work is that no one discipline is capable of covering it all. Experimental physics, theoretical physics, computer science, mathematics—each and every one is involved to the same extent.”
To find out more about quantum science at Erlangen, including current opportunities, please contact Prof. Dr. Joachim von Zanthier, Prof. Dr. Michael Hartmann, or PD Dr. Christoph Marquardt.
Structured doctoral programs are offered by the International Max Planck Research School for the Science of Light and the Max Planck School of Photonics.